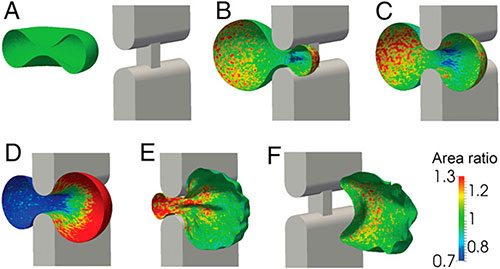
Computer models developed at Brown show how red blood cells move through tiny slits in the spleen. The work suggests that the limitations imposed by these slits determine the size of red blood cells.MIT/CMU/Brown
PROVIDENCE, R.I. [Brown University] — A key function of the spleen, a fist-sized organ located just behind the stomach, is to act as a blood filter, taking malformed or damaged red blood cells out of circulation.
But a new paper in the Proceedings of the National Academy of Sciences suggests that the spleen also plays a primary role in determining the size of red cells, making sure they are small enough to pass safely through the tiniest capillaries in the body. The work not only suggests an underappreciated role for the spleen in shaping cells, it could help in developing drugs for diseases such as malaria that affect the size and shape of red blood cells.
The research, which was led by scientists from MIT and Carnegie Mellon, drew heavily on computer models developed in the lab of George Karniadakis, professor of applied mathematics at Brown. Karniadakis, a co-author on the new paper, discussed the findings and his modeling approach.
Could you give us a few details about the new aspect of the spleen’s function this research discovered?
We have simulated the process of red blood cells (RBCs) flowing through the tiny slits in the spleen through which blood is filtered. It’s not possible to observe this in humans, so we wanted to use simulation to quantify those dynamics. These slits are smaller than even the smallest capillaries—less than three microns (millionths of a meter)—and hence this narrow clearance imposes a very stringent mechanical deformation for RBCs. In particular, our simulations have revealed a unique relationship for the human RBCs that defines which ones will go through the spleen and which ones will not. It is the first such study of the biomechanical interactions of the spleen and RBCs.
Can you explain a little about how the models used in this study were developed?
The models used for his study have been developed over the last 10 years at Brown University by several PhD students and postdocs, starting with the PhD thesis at Brown of Igor Pivkin, who is the first author of this paper. They rely on a method called Dissipative Particle Dynamics or DPD, in which we systematically estimate the molecular dynamics of soft matter and complex fluids.
How were the models tuned to investigate the spleen?
We developed DPD models for individual red blood cells, as well as whole blood both in health and disease. The models have been informed and validated by experimental data from the literature and from experiments conducted by our MIT collaborators in the nanomechanics lab of Subra Suresh and Ming Dao. In particular, David Quinn, a former Ph.D. student in the Nanomechanics lab, designed specific microfluidic experiments to produce narrow slits of 2 microns by three microns. Those opening are similar to the splenic slits, and the results from those experiments were used to calibrate the DPD-based models.
How might this finding contribute to potential treatment strategies for malaria?
The spleen plays a particularly important role in malaria patients, as malaria-infected RBCs are stiff due to the presence of the parasite inside the RBC. These stiffer RBCs may cause spleen enlargement and our model can help quantify this process.
Could you talk a little bit about how these bloodflow models have been applied in the past?
We have also validated our DPD-based methodology in a number of hematological disorders, both inherited or due to infection. For example, our models have shown how different cell types interact to cause bloodflow blockages associated with sickle cell anemia. We’ve also used the models to show how malaria-infected cells impede bloodflow. This has been work we’ve done jointly with our MIT collaborators supported by NIH.