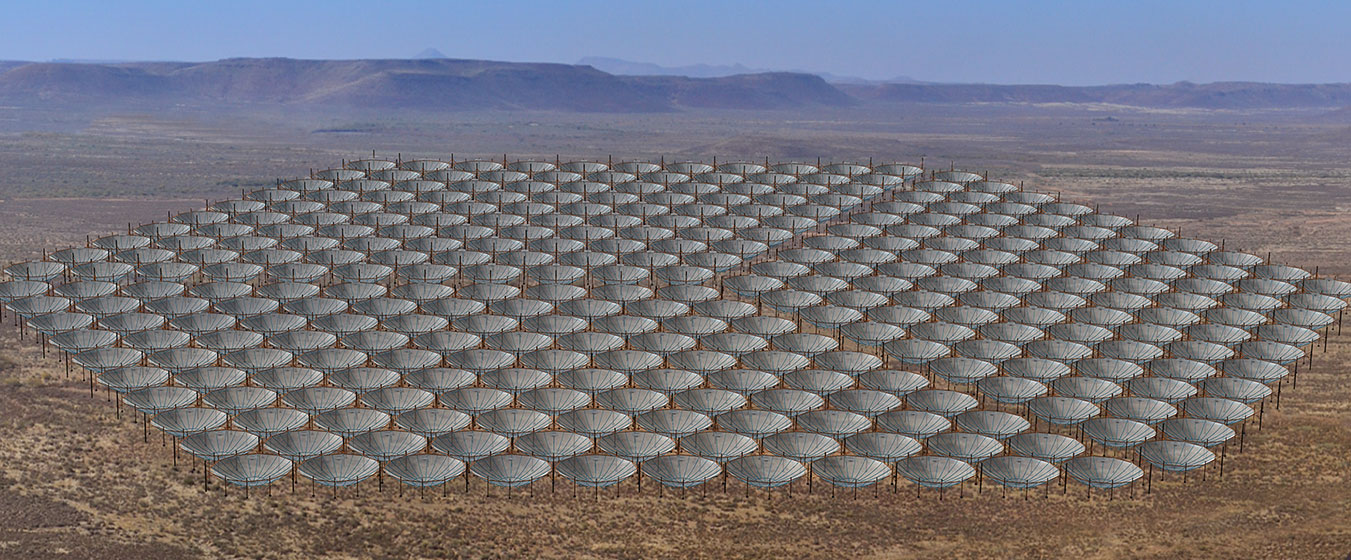
When completed in 2018, the HERA telescope will have an array of 240 radio antennae, scanning the sky for clues about the first stars and galaxies in the universe.
PROVIDENCE, R.I. [Brown University] — An experiment that will peer deep into the past to a time when stars and galaxies first illuminated the universe is getting a nearly $10 million boost from the National Science Foundation.
The experiment, an international collaboration called the Hydrogen Epoch of Reionization Array, or HERA, currently has 19 radio dishes, each 14 meters wide, aimed at the southern sky near Carnarvon, South Africa. The $9.5 million in new funding will expand that array to 240 dishes by 2018.
HERA will look across the universe and more than 13.5 billion years back in time, to the period when the first stars began producing light, creating bubbles of ionized hydrogen gas around them. Those bubbles expanded outward, slowly filling all interstellar space with the ionized hydrogen we see today. By searching for the faint signal of that hydrogen ionization process, HERA scientists hope to learn more about the period when the universe first became bathed in light and what those first stars and galaxies were like.
The effort is led by the University of California, Berkeley, with partner institutions including Brown, University of Washington, UCLA, Arizona State University, the National Radio Astronomical Observatory, the University of Pennsylvania, the Massachusetts Institute of Technology, Brown University, the University of Cambridge in the UK, the Square Kilometer Array in South Africa and the Scuola Normale Superiore in Pisa, Italy.
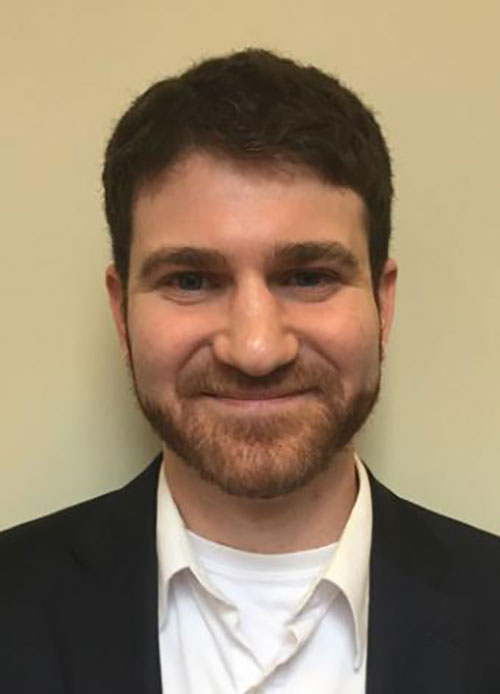
"We’ll be documenting a cornerstone event in cosmic history."
Jonathan Pober, assistant professor of physics at Brown, is a member of the HERA science team. He talked about the experiment in an interview.
Can you explain more about the period in the universe’s history that HERA is investigating?
We’re looking at this period of reionization, which happened about 500 million years after the Big Bang. Thinking back to just minutes after the Big Bang when the first hydrogen atoms formed, they were all ionized — there was so much energy around that protons and electrons were stripped from each other. As the universe cooled and expanded, protons and neutrons started to recombine, which slowly filled the universe with neutral hydrogen. But around 500 million years after the Big Bang, all of the hydrogen in the universe outside of galaxies — in what we call the intergalactic medium — got reionized. It’s still that way today.
Our best model for what caused this reionization is that when luminous matter — stars and black holes and galaxies — starting forming, they put energy back into the universe that reionized the surrounding hydrogen gas. The picture we have is like blowing bubbles. Light from these first stars and galaxies made bubbles of ionized hydrogen, which grew outward. Eventually they all overlap and we get the completely ionized intergalactic medium we see today. We want to know more about this reionization period, which is ultimately tied to questions about what the first stars and galaxies were like. Were they putting out more ultraviolet photons, more x-ray photons or what? How were the first stars formed? Did they live a long time or were they blips in the night? These first stars and galaxies are the building blocks of everything we see today, so we’d like to understand what they were like.
What signal from the reionization epoch is the experiment looking for?
These galaxies are too faint to see the light from them directly. So HERA is a radio telescope that looks for a signal from neutral hydrogen. We’re looking back to the time when ionization was still happening. Neutral hydrogen emits radiation at a wavelength of 21 centimeters, and that’s the signal we’re tracking. As reionization happens, and these bubbles of ionized hydrogen start growing, the 21-centimeter signal of neutral hydrogen decreases. So we’re effectively looking for the disappearance of this signal.
Because the universe has been expanding for that last 13 billion years, the 21-centimeter signal we’re looking for will be stretched out, and the amount that it’s stretched tells us when in time it was emitted. The more stretched it is, the older it is. The signal we’re looking for will be stretched to about two meters.
What does the telescope look like?
It’s an array of radio antennas — dishes about 14 meters across each. We have 19 of these right now, and this new grant will get us to 240. So when it’s done it will be about three football fields across. We’re in the Karoo Desert in South Africa, probably the most desolate place I’ve ever been. But that keeps us away from a lot of radio interference.
I like to describe this as a software telescope. Each of those antennas feeds back to a supercomputer, which correlates every antenna to every other antenna. Each pair of antennas is sensitive to a particular scale in the sky. You use those cross correlation to reconstruct information about the sky.
The hardware is pretty simple. If you’re looking at the optical wavelength, you polish your mirror to nanometers. But we’re looking wavelengths on the order of meters, so we can build our antennas out of PVC pipe and chicken wire. And that’s what we did. At those wavelengths, chicken wire is a pretty good mirror.
What’s your role in the collaboration?
The signal we’re looking for is really faint, so we need a bevy of tools for discriminating that signal from all the other stuff we might be seeing. I’m a data analyst, so I’ll be working on the end-to-end simulation of the data. We’re simulating the data from scratch, so we know what it will look like coming out of the telescope with all the confounding effects. That way we can make sure we understand what we’re getting out of the experiment.
When we detect a signal, and I truly hope we will, it’s going to take a lot of convincing — both internally within the experiment and externally — that the signal is real, so that’s what we’ll be focusing on at Brown.
And if we can confirm that signal, we’ll be documenting a cornerstone event in cosmic history.