A semester-long program at ICERM and a dynamic new hire at Carney promise a major payoff for brain science
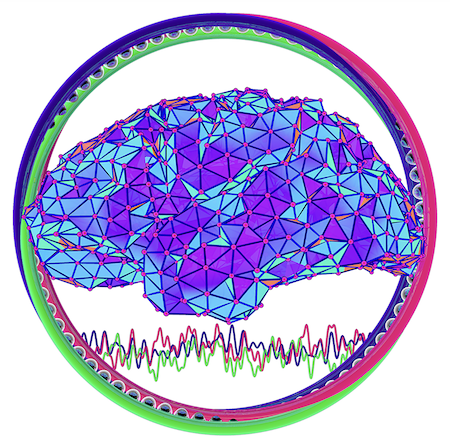
Mathematical neuroscientist Carina Curto led an ambitious semester program at ICERM this fall, attracting neuroscientists and mathematicians from all over the world. She brings her vision to Carney in July 2024.
Experimental neuroscientists who are employing topology to drive their research of the brain’s visual, olfactory and auditory systems. Pure mathematicians who are thinking about applying geometric tools to neuroscience. Computational neuroscientists who have run into mathematical bottlenecks in their work.
More than 300 mathematicians and neuroscientists traveled to Providence during the fall of 2023, drawn by the opportunity to learn about and discuss these kinds of fascinating intersections between math and brain science. Between September 6 and December 8, each of these participants played a part in bridging the divide between their fields in order to speed progress in brain science research.
“Math+Neuroscience: Strengthening the Interplay between Theory and Mathematics,” was an ambitious semester-long program backed by the Institute for Computational and Experimental Research in Mathematics (ICERM), an NSF-funded math institute at Brown University. “Math+Neuroscience” featured three weeklong workshops across the fall calendar and sponsored sixty-six researchers who spent the entire semester collaborating at ICERM. Together, the workshops and semester cultivated a rich environment where neuroscientists and mathematicians could connect.
Carina Curto.
The lead co-organizer of the semester program was Professor Carina Curto, a mathematical neuroscientist who will join Brown and Carney as a Professor of Applied Mathematics and Brain Science in the summer of 2024. On Monday, October 16, she stood in front of the floor-to-ceiling whiteboard in ICERM’s packed auditorium, launching the weeklong workshop dedicated to topology and geometry in neuroscience, an area that is one of her specialties.
Addressing the neuroscientists in the room, Curto said, “If you can turn the problem into a concrete mathematical question that doesn’t require a deep and sophisticated understanding and years of training in neuroscience, mathematicians will work on it.”
Her statement was the semester-long program’s thesis. In designing the program, Curto and co-lead organizer professor Katie Morrison (University of Northern Colorado) were inspired by the notion in mathematics of “open problems”—unsolved mathematical challenges like the famed Millennium Prize Problems that are made available to the entire math community for any interested mathematician to tackle. The semester’s priority was to first identify and then compile the mathematical gaps and problem areas neuroscientists are encountering in their research.
“Neuroscience is very exciting right now, and there are many mathematicians who are interested, but they don’t know where their input could be useful,” Curto said, explaining the rationale behind the semester.
“Meanwhile, on the neuroscience side, a lot of the technological bottlenecks to further progress are mathematical. Beyond just our need to deal with larger datasets, we also need to develop mathematically informed theories that do justice to the complexity of the brain and to the results we are seeing in our experiments. I see collecting open problems as a way to build some bridges.”
Curto, Morrison and their eight other co-organizers collected neuroscience open problems through a combination of research presentations, group projects and conversations. Most importantly, “open problem sessions”–blocks of time throughout the semester where an organizer catalyzed group discussion – helped participants to refine and assess the open questions that surfaced during a given week’s many events. Later this year, Curto and her co-organizers will make the open problems they collected publicly accessible–a practice they believe has the potential to speed up research in theoretical neuroscience.
When Curto joins Brown officially in July, she will bring her finely tuned ability to formulate and crack into these open problems, opening up a wealth of possibilities for training and collaboration. "Professor Curto’s keen capacity to lend insight into many areas of brain science is really exceptional,” said Michael Frank, director of Carney's Center for Computational Brain Science and chair of the committee that hired Curto. “We are very excited about how her collaborative spirit and mathematical chops will inspire new directions for Carney.”
A LAND OF IMMIGRANTS
Curto’s path into neuroscience was not a straightforward one. She earned her A.B. in physics at Harvard and her Ph.D. in mathematics at Duke and was working on string theory when research around place cells and grid cells exploded. (Place cells and grid cells are neurons that become active as an animal moves around in space.) Fascinated with the prospect of what further discoveries math could bring to neuroscience, Curto traded the possibility of continued math and physics specialization for a postdoc doing computational neuroscience in a lab side-by-side with experimental neuroscientists.
In addition to what she learned about the nuts and bolts of neuroscience, Curto also saw that the field is, by necessity, one that borrows from many other disciplines in order to work with the brain in all its complexity. “Neuroscience is like a land of immigrants,” she said. “I'm an immigrant from the math community.”
As Curto’s postdoctoral training developed her ability to analyze and model complex nonlinear neuroscience data, her knowledge of math techniques from algebraic geometry, combinatorics and algebraic topology–quite literally–enabled her to reveal geometric structures within that data. Notably, in 2015 Curto and her co-authors introduced a novel method called clique topology to enable neuroscientists to detect geometric organization in neural activity from the activity data alone. This method has enabled subsequent research to uncover the structure of the brain’s olfactory space.
Following this work, Curto and Morrison began working on neural network theory, examining how different kinds of nonlinear dynamics emerge from network architecture. An article the duo published in 2023 presented a theory that uses graph rules and combinatorics to predict attractors in a neural network. This work reveals the important role of the graph of connectivity among neurons in shaping neural activity and computation.
While the time and effort involved in completely retraining in neuroscience is not something every mathematician can take on, Curto knows from her own experience that making the language of brain science just a bit easier to interpret can have a huge impact. “What I am trying to do,” she explained, “is find ways to make neuroscience more accessible to facilitate interactions with my old-world community. And I’m not trying to do it alone.”
Professor Brendan Hassett, ICERM’s director and a pure mathematician, witnessed how effective Curto is at bridging the two disciplines first hand. ICERM, he said, wanted to offer a program like Math + Neuroscience for some time. Its Scientific Advisory Board was elated when Curto and her co-organizers proposed a program that captured the breadth of what different mathematical techniques can offer a wide range of neuroscience problems.
This is a daunting undertaking, because both fields contain so many sub-specialities. Just as there are different flavors of neuroscience (cognitive, behavioral, molecular and so forth), the field of mathematics contains a rich array of approaches. Algebraists, topologists, analysts and combinatorialists all think about different kinds of questions and tackle them in different ways.
“Math+Neuroscience” took on this challenge by breaking apart the semester into three major neuroscience themes that various mathematical approaches could address. The semester’s first weeklong workshop considered how dynamical systems, statistical mechanics, linear algebra and geometry can help answer questions about how network connectivity shapes neural activity. The second week considered how applied topology and geometry can function as a useful language for understanding how the brain’s sensory systems work. And the third week explored how algebra, combinatorics, probability and geometry can help “crack the neural code,” or decode how populations of neurons send and transform messages to each other.
“I am thrilled at the range of mathematics represented at this program!” Hassett said.
According to Curto, the time is right for this rich exchange between the two fields to happen and to pay off big. “I’m inspired by my background in theoretical physics. In the 20th century, you saw huge advances because physicists and mathematicians had enough of an ability to communicate that they could pass the problems they were working on back and forth. In computational and theoretical neuroscience, many of us now have enough of a shared language with mathematics that we can do that too. I think we are at a point where there’s critical mass and it’s going to help advance the field.”
In addition to Katie Morrison (University of Northern Colorado), Math + Neuroscience’s co-organizers included Brent Doiron (University of Chicago), Robert Ghrist (University of Pennsylvania), Kathryn Hess (EPFL), Zachary Kilpatrick (University of Colorado Boulder), Matilde Marcolli (California Institute of Technology), Konstantin Mischaikow (Rutgers University), Elad Schneidman (Weizmann Institute of Science), and Tatyana Sharpee (Salk Institute).